Introduction
In the last sixty years, the freshwater demand has more than doubled and as many as 3.5 billion people could experience water scarcity by 2025. Agriculture is the largest global user of freshwater and its consumption has increased by a 100 % in the last century while industrial water demand has more than tripled, due to an increasing requirement of electricity, fuel and water-intensive goods like textiles. During the same period, domestic water use has quickly risen by 600 %1. As the world population is still growing fast, finding suitable technologies to achieve a smarter water reuse and reduce our water footprint is urgent and of vital importance.
Hard time for conventional water treatment processes
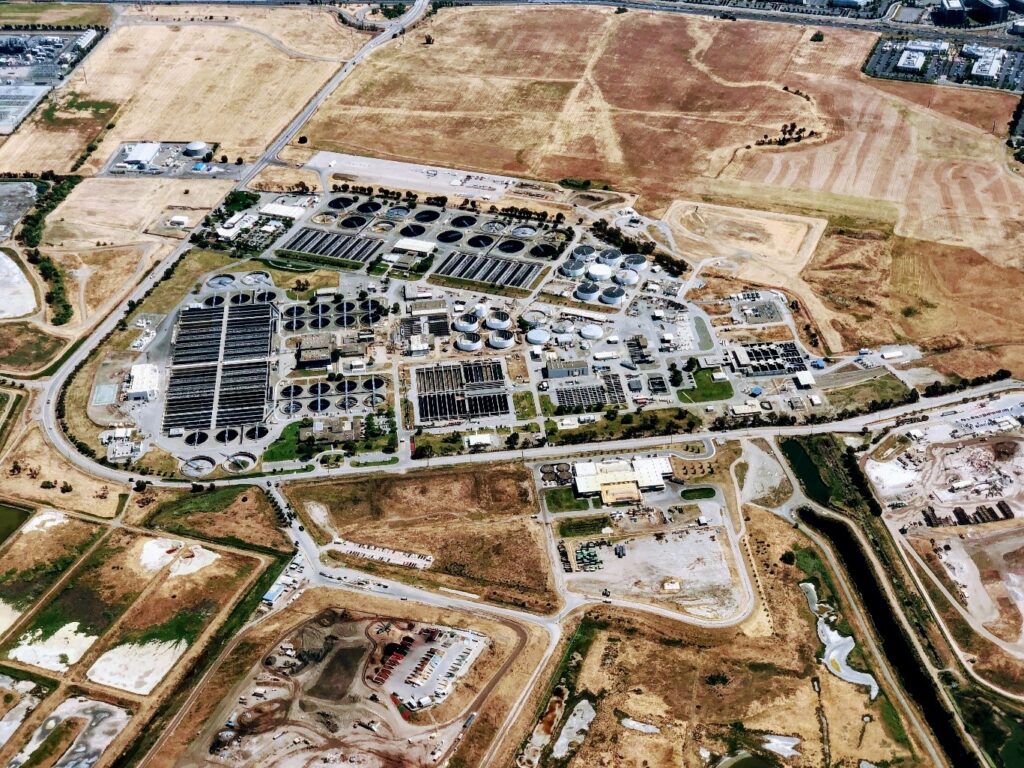
According to the WHO, 500 million people die each year because of poisoning or disorders associated to water contamination by biological or chemical agents3. The need to tackle water scarcity together with health issues related to pollution calls for the introduction of treatment capability beyond conventional water treatments methods. Water contaminants may include organic and inorganic substances, which can be toxic, and pathogens. Conventional water treatment processes are not always very effective in their removal. For instance, coagulation, sedimentation and filtration units are unable to effectively remove trace organic compounds such as pharmaceuticals, personal care products and endocrine disrupting chemicals4. Moreover, chlorination can lead to the formation of by-products such as trihalomethanes (e.g. chloroform), haloacetic acids and chloramines, which can be even more toxic than the parental molecules and thus requiring further depuration steps5. In order to meet the modern requirements for water quality, a combination of reverse osmosis and advanced oxidation processes (AOPs) has been often proposed. Moreover, UV irradiation is typically added as an additional layer of protection against pathogens and some chemicals. However, treatment costs are typically higher than conventional methods6.
Advanced oxidation processes (AOPs)
AOPs refer to those processes that generate large amounts of OH radicals, which have proven to be effective in the decomposition of a wide range of organic contaminants, toxins and pathogens. Conventional AOPs generally involve the addition of chemical precursors on site as well as adequate infrastructure to accommodate storage. Therefore, treatment costs exceed that of conventional water treatment methods, making them less attractive for the commercial scale and poorly accessible to the underdeveloped regions of the world, where the water scarcity is more severe6.
Plasma is here to help
At this purpose, plasma technologies are attracting more and more interest as a source of advanced oxidation species. Plasma-liquid interactions can offer a number of features that conventional treatment cannot provide at all and conventional AOPs can only provide at high cost. In particular, Foster6 points out that plasma can be generated in regular air or in the liquid itself, without the requirement of consumables. Moreover, plasma can combine the effects of different reactive species simultaneously, leading to faster decomposition rates. In addition, its application is inherently modular and therefore can be easily suited in a conventional water treatment system to substitute and reduce the number of depuration steps6.
Plasma for organic contaminant reduction
The reliability of plasma treatment to decontaminate water from organic pollutants has been deeply investigated in the past years. Magureanu et al.7 reviewed numerous studies on the removal of harmful pollutants such as phenols, organic dyes, pharmaceuticals and pesticides. While the potential of plasma to remove these contaminants is widely accepted, many efforts are still required to improve the energy efficiency of their decomposition and mineralization. In this respect, reactor design stands out as a major critical point. Besides the intrinsic characteristics of the pollutants and the aqueous medium, faster and efficient removal rates depend on the mass transfer of reactive species from the plasma to the liquid. At this purpose, maximizing the contact area between the plasma and the treated solution is of crucial importance. This could be achieved by generating a foam on the liquid surface or spraying the solution through the discharge area7,8. Magureanu et al.7 also points out that the combination of plasma treatment with other AOPs can enhance the removal efficiency by the generation of additional OH radicals. This can be realized either by recycling the effluent gas from plasma, rich in ozone, or by adding a catalyst to promote Fenton oxidation with the plasma-generated hydrogen peroxide. Indeed, additional ozone gas bubbling could improve removal rate of alachlor, an aromatic pesticide, and energy cost by more than 50 %9. Nevertheless, as much efficient as it is considered compared to conventional processes, plasma is energy consuming. At this purpose, Jiang et al.10 suggested that biological treatment should be coupled with plasma into integrated technologies for water treatment. Here, the AOP treatment step should be minimize as much as possible in favor of the biological stage, which is almost energy free. Therefore, the role of plasma should be limited to the breakdown of non-biodegradable molecules into biodegradable byproducts, leaving the task of completing the decomposition and mineralization processes to the biological treatments.
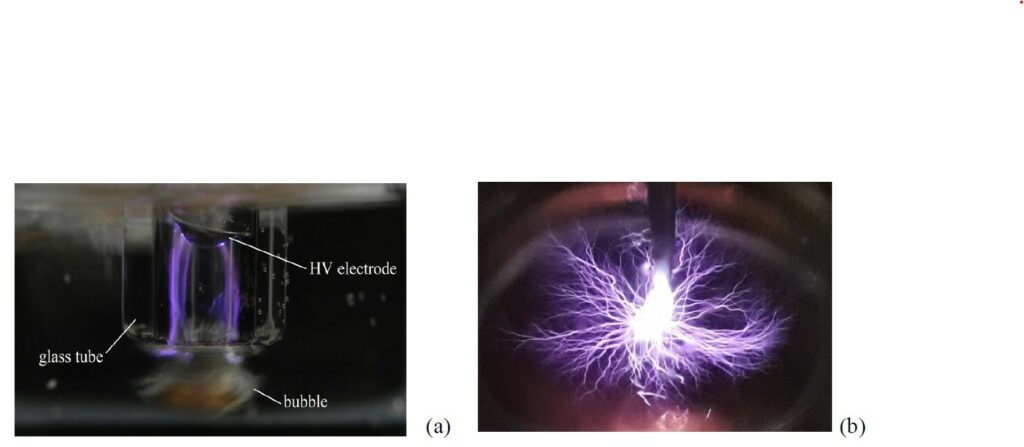
Perfluoroalkyl substances: a new threat to water quality
Unfortunately, there are some extreme situations where most of the work cannot be delegated the biological treatment step and almost all the mineralization process needs to be completed beforehand. This is the case of perfluoroalkyl substances (PFAS), whose degradation byproducts are still harmful and non-biodegradable. PFAS are a group of more than 4700 chemicals and are used in a wide variety of consumer products and industrial applications because of their unique chemical and physical properties, including oil and water repellence, temperature and chemical resistance and surfactant properties. Those outstanding features are deriving from the fluoro-carbon bonds, which are extremely stable and thus unreactive. Their extraordinary characteristics turn out to be very undesirable when it comes to remove PFAS from water. The high hydrophobicity of the perfluorinated tails makes PFAS very prone to bioaccumulation and therefore potentially dangerous even at very low concentrations. On the other hand, their surfactant behavior and persistency bring those molecules far away from the source of contamination. Detection of PFAS in water is still very challenging and requires advanced analytical methods such as high-resolution mass spectrometry. To face this new challenge, water treatment plants are often equipped with activated carbon beds and ion exchange resins to adsorb PFAS. However, these techniques are affected by short breakthrough times and generate waste products, which require further treatments13. Moreover, most of the AOPs, based on oxidation by OH radicals, have proven to be inefficient against PFAS14. At this purpose, plasma technologies can be helpful to handle the issues related to the removal of these hazardous molecules by featuring a wide range of reactive species beyond OH radicals. Of particular interest are aqueous and free electrons, which are able to attack and remove fluorine from the long perfluorinated tails protruding from the solution surface into the gas phase. When the plasma feed gas is argon and a positive voltage polarity is applied, argon ions may also initiate reactions of charge transfer and trigger the decomposition of PFAS15. Moving from air to argon as feed gas can indeed improve the energy efficiency and lead to a faster mineralization without producing nitric acid and thus maintaining almost unaltered the characteristics of the aqueous medium11. Moreover, argon allows recirculation of argon through the reactor, reducing its consumption and eventually leading to decomposition of gaseous by-products which otherwise would be introduced in the atmosphere. Here, reactor design is extremely important and should focus on increasing the contact area between plasma and solution. In addition, bubbling should be introduced in order to increase the concentration of PFAS in the plasma-liquid interface11,15.
New challenges for plasma: micro- and nanoplastics
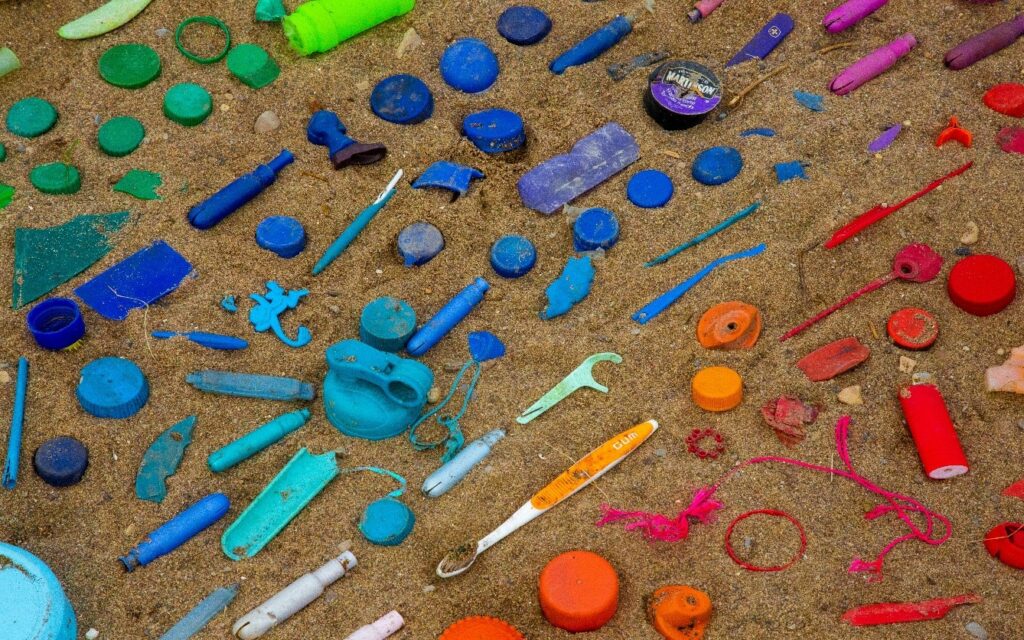
After disposal, up to 70 % of plastics is lost to the environment. Most of plastic materials are hardly degradable through weathering and ageing, thereby accumulating in the aquatic system for decades. Their slow degradation leads to the formation of plastic debris of different size. Particles with a diameter between 1 and 5 µm are defined microplastics (MPs), while particles with lower diameters are categorized as nanoplastics (NPs). Water and wastewater treatment plants are considered as an important pathway for the release of NPs/MPs since fragmentation and ageing of plastics can be accelerated by photo-oxidation by UV light, hydrolysis and mechanical fracture17. MPs are also contained in some commercial products such as face cleaners, drilling fluids, 3D printing products, pharmaceutical vectors and industrial abrasives and thus are directly introduced in the environment18. Detection of NPs/MPs is still very challenging and, due to their small particle size, only a few techniques can give a reliable quantification. Those techniques would be Raman and micro-Raman spectroscopy dynamic light scattering (DLS) and nanoparticles tracking analysis (NTA) which are quite expensive and sensitive to impurities in real water and wastewater samples17. AOPs, such as photo-degradation by UV light and chemical oxidation, have been proposed as suitable way to reduce the concentration of NPs/MPs in water but they are limited by long process periods, making them disadvantageous for scaling up18. However, ozonation led to formation of carbonyl groups on the surface of polystyrene plastic making its mineralization by microorganisms faster. Moreover, the generation of reactive oxygen species and strong UV irradiation in plasma systems could work together to induce the dissociation of C-C and C-H bonds in the PVC MPs19. In recent years, a few studies to simulate the artificially-accelerated aging of MPs have been carried out and those are reviewed by Liu et al.20 The aging potential of plasma treatment compared to the other processes tested is outstanding and very promising. However, no studies have been conducted on the possibility to decompose and/or make NPs/MPs more biodegradable by plasma treatment so far.
To conclude…
Plasma is a very promising and versatile tool, which can help to tackle water scarcity and reduce the disparity on the access to high quality drinking water. The wide range of reactive species produced can simultaneously decontaminate water by organic and inorganic compounds, pathogens and perhaps even by micro and nanoplastics, with a low energy demand and cost. Studies on the upscaling of such technologies must be prioritized as the stress on the water resources is constantly increasing.
This text was written by Omar Biondo.
References
1World Resources Institute. (2021, March 9). Retrieved from https://www.wri.org/
2Aerial view of a wastewater facility in California, US [online]. Available at: [https://unsplash.com//][Accessed 11 March 2021]
3World Health Organization (WHO). (2018). Retrieved from http://www.who.int/
4Huerta-Fontela, M., Galceran, M. T., & Ventura, F. (2011). Occurrence and removal of pharmaceuticals and hormones through drinking water treatment. Water Research 45, 3, 1432-1442.
5Gopal, K., Tripathy, S. S., Bersillon, J. L., & Dubey, S. P. (2007). Chlorination byproducts, their toxicodynamics and removal from drinking water. Journal of Hazardous Materials 140, 1-6.
6Foster, J. E. (2017). Plasma-based water purification: Challenges and prospects for the future. Phys. Plasmas 24, 055501.
7Magureanu, M., Bradu, C., & Parvulescu, V. I. (2018). Plasma processes for the treatment of water contaminated with harmful organic compounds. J. Phys. D: Appl. Phys. 51, 313002 (23pp).
8Stratton, G. R., Bellona, C. L., Dai, F., Holsen, T. M., & Mededovic Thagard, S. (2015). Plasma-based water treatment: Conception and application of a new general principle for reactor design. Chemical Engineering Journal 273, 543-550.
9Wardenier, N., Gorbanev, Y., Van Moer, I., Nikiforov, A., Van Hulle, S. W., Surmont, P., . . . Vanraes, P. (2019). Removal of alachlor in water by non-thermal plasma: Reactive species and pathways in batch and continuous process. Water Research 161, 549-559.
10Jiang, B., Zheng, J., Qiu, S., Wu, M., Z. Q., Yan, Z., & Xue, Q. (2014). Review on electrical discharge plasma technology for wastewater remediation. Chemical Engineering Journal 236, 348-368.
11Saleem, M., Biondo, O., Sretenović, G., Tomei, G., Magarotto, M., Pavarin, D., . . . Paradisi, C. (2020). Comparative performance assessment of plasma reactors for the treatment of PFOA; reactor design, kinetics, mineralization and energy yield. Chemical Engineering Journal 382, 123031.
12Tomei, G. (2019). Plasma non-termico per la degradazione di acido perfluoroottanoico (PFOA) in acqua (Master’s thesis). Università degli studi di Padova, Italy.
13Woodard, S., Berry, J., & Newman, B. (2017). Ion exchange resin for PFAS removal and pilot test comparison to GAC. Remediation 27, 19-27.
14Trojanowicz, M., Bojanowska-Czajka, A., Bartosiewicz, I., & Kulisa, K. (2018). Advanced Oxidation/Reduction Processes treatment for aqueous perfluorooctanoate (PFOA) and perfluorooctanesulfonate (PFOS) – A review of recent advances. Chemical Engineering Journal 336, 170-199.
15Stratton, G. R., Dai, F., Bellona, C. L., Holsen, T. M., Dickenson, E. R., & Mededovic Thagard, S. (2017). Plasma-Based Water Treatment: Efficient Transformation of Perfluoroalkyl Substances in Prepared Solutions and Contaminated Groundwater. Environ. Sci. Technol. 51, 1643-1648.
16Collected plastic during Community Cleanup at the shoreline and harbourfront in Hamilton, Canada [online]. Available at: [https://unsplash.com//][Accessed 11 March 2021]
17Enfrin, M., Dumee, L. F., & Lee, J. (2019). Nano/microplastics in water and wastewater treatment processes – Origin, impact and potential solutions. Water Research 161, 621-638.
18Rodríguez-Narvaez, O. M., Goonetilleke, A., Perez, L., & Bandala, E. R. (2021). Engineered technologies for the separation and degradation of microplastics in water: A review. Chemical Engineering Journal, 128692.
19Zhou, L., Wang, T., Qu, G., Jia, H., & Zhu, L. (2020). Probing the aging processes and mechanisms of microplastic under simulated multiple actions generated by discharge plasma. Journal of Hazardous Materials 398, 122956.
20Liu, P., Shi, Y., Wu, X., Wang, H., Huang, H., Guo, X., & Gao, S. (2021). Review of the artificially-accelerated aging technology and ecological risk of microplastics. Science of the Total Environment 768, 144969.